Medical Brief: Melanoma Understanding the DNA Mutation Landscape
Melanocytic tumors are a relatively common group of neoplasms in dogs that originate from a pigment producing cell or melanocyte from the epidermis, dermis or hair follicles. Melanomas are the most common type of melanocytic tumor in the dog and affects approximately 19,000 dogs annually in the US, representing 7% of all malignant tumors in this species. Melanomas occurs in middle-aged to older dogs with mean age of 11.6 years, with no sex predisposition. Some breeds are more likely to develop melanoma in certain locations than others suggesting genetic factors are involved in melanoma development. The exposure to ultraviolet light is not a common etiology for most canine melanomas, as they occur predominantly in the mouth, at the nail bed, or on the skin which is typically covered by hair. This situation is similar to that for mucosal melanomas in people 1–5.
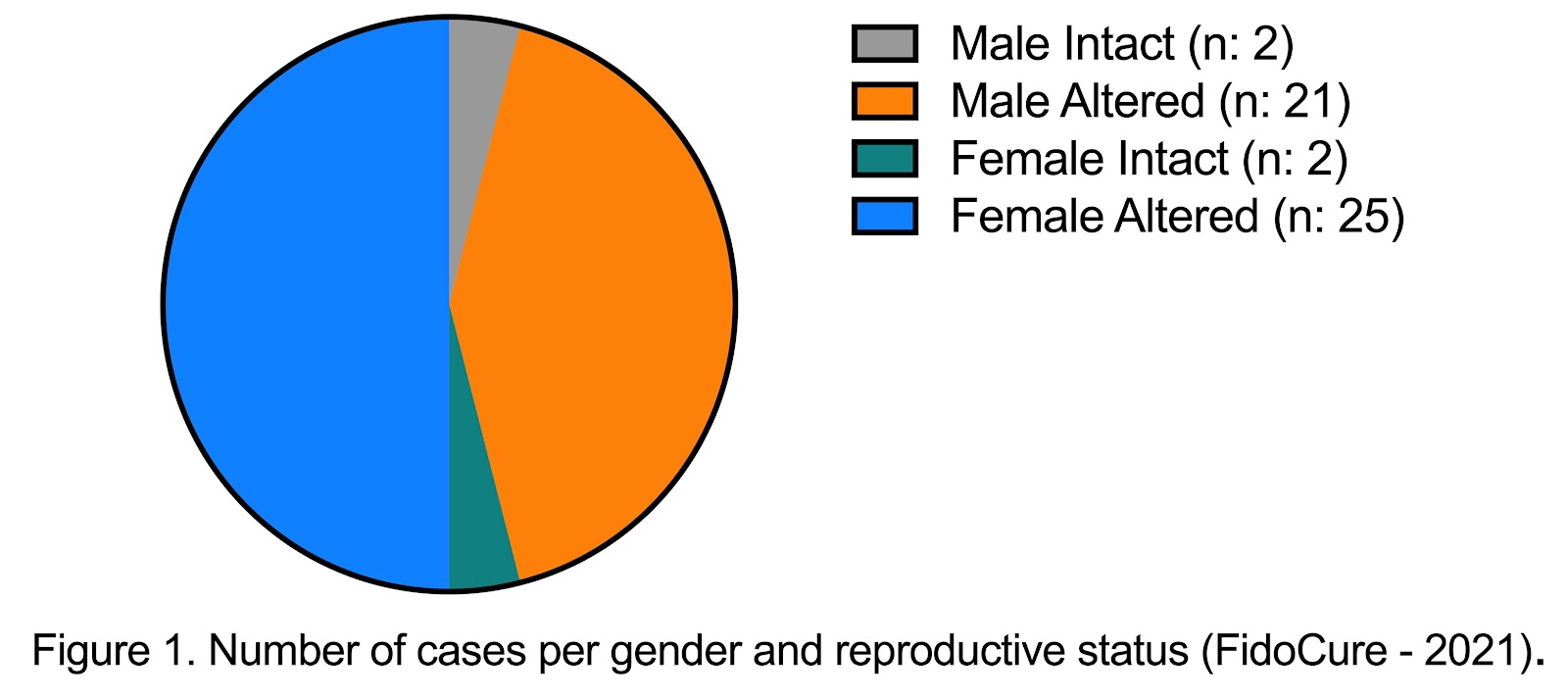
Corresponding to the literature, the analysis of melanoma tumors submitted to the FidoCure® Platform for DNA sequencing reveal equal gender distribution (Figure 1) and a mean age of 10.87 years old. The most common presentation of melanomas in dogs is in the oral cavity, followed by the skin, nail bed, and eyes. This is similar to the cases submitted to FidoCure® for DNA sequencing: 34 mucosal, 10 cutaneous, 2 digit, 1 ocular, and interestingly 5 cases of visceral melanomas. The anatomical localization of the primary tumor is often correlated with prognosis. Mucosal melanomas are considered to have the worst prognosis followed by the digital and cutaneous forms. Oral mucosal melanomas are very aggressive, being both locally invasive and having a high metastatic rate to both regional lymph nodes and the lungs. Affected dogs have clinical signs that are often associated with both the clinical stage and location of the tumor. For example, dogs with oral melanomas frequently display dysphagia, halitosis, ptyalism, and oral bleeding 3,6,7. The median survival time (MST) for dogs with oral melanoma is around 6 months, while it is 11.8 months for dogs with cutaneous melanomas 2,6.
The preliminary diagnosis of canine melanomas can be obtained by fine-needle aspiration (FNA) and cytology analysis. Histopathology and immunohistochemistry confirm the diagnosis and provide important information about the prognosis. The immunostaining of Ki67, a biomarker indicating cellular proliferation, is useful to predict the biologic behavior of both oral and cutaneous melanomas. Thoracic radiographs, CT scans and/or MRI scans are used to determine the clinical stage and to investigate the invasiveness of the primary tumor, the integrity of the bones of oral and nasal cavity in cases of oral melanomas, the presence or absence of lung metastases and whether non palpable lymph nodes are enlarged 6,8,9.
The choice of treatment for melanomas in dogs is affected by several parameters, such as location, invasiveness and the clinical condition of the pet. Surgical excision and radiation therapy are potential therapeutic options for the local disease. Surgical excision is often reserved for smaller, less invasive tumors that can be removed with complete margins, or for dogs that do not have access to radiation therapy. For invasive tumors and/or large tumors that cannot be completely resected, radiation therapy is often the best form of local therapy. Similarly, when the melanoma affects the digit, surgical amputation of the affected digit or digits is often the recommended local therapy. If the digit melanoma is too invasive or large and cannot be resected completely, then radiation therapy is an option for local disease control. Radiation therapy, although effective at controlling local disease in patients with stage II and III disease, does not address the metastatic nature of malignant melanomas in dogs 2,6,10–15. Systemic therapy in the form of chemotherapy, (eg carboplatin), electrochemotherapy and COX-2 inhibitors are often recommended to address the systemic nature of this disease 6,16–2. Due to many melanomas being chemoresistant, the efficacy of adjuvant chemotherapy to treat melanoma is limited. Immunotherapy is another systemic adjuvant treatment option for dogs with melanoma. The Xenogeneic DNA vaccine, OnceptⓇ, may increase overall survival when used in certain dogs with melanoma, either oral or digital 2,6,21–25.
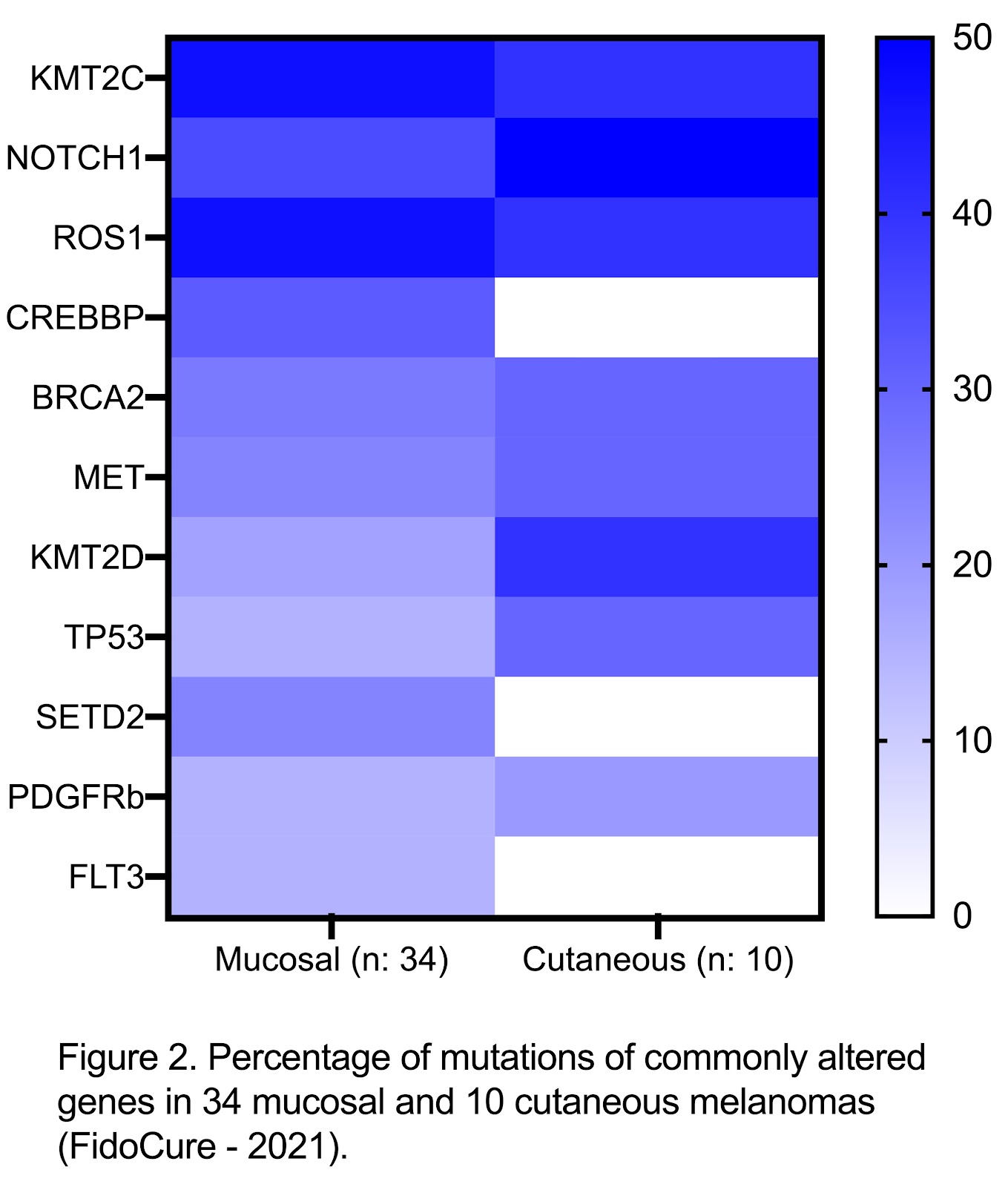
Although the development of these adjuvant therapies has partially improved outcomes in dogs with melanomas, tumor heterogeneity and resistance mechanisms are still challenges and more effective therapies are needed, especially in dogs with metastatic disease. Genomic studies to discover novel mutations in canine melanomas are underway, allowing us to look for molecular markers associated with prognosis and potential therapeutic targets 2,26. Based on the FidoCure® data analysis, mucosal melanomas have a higher percentage of mutations compared to cutaneous melanomas (Figure 2). This contrast in the mutational landscape may contribute to the difference in the biologic behavior of these two types of melanoma.
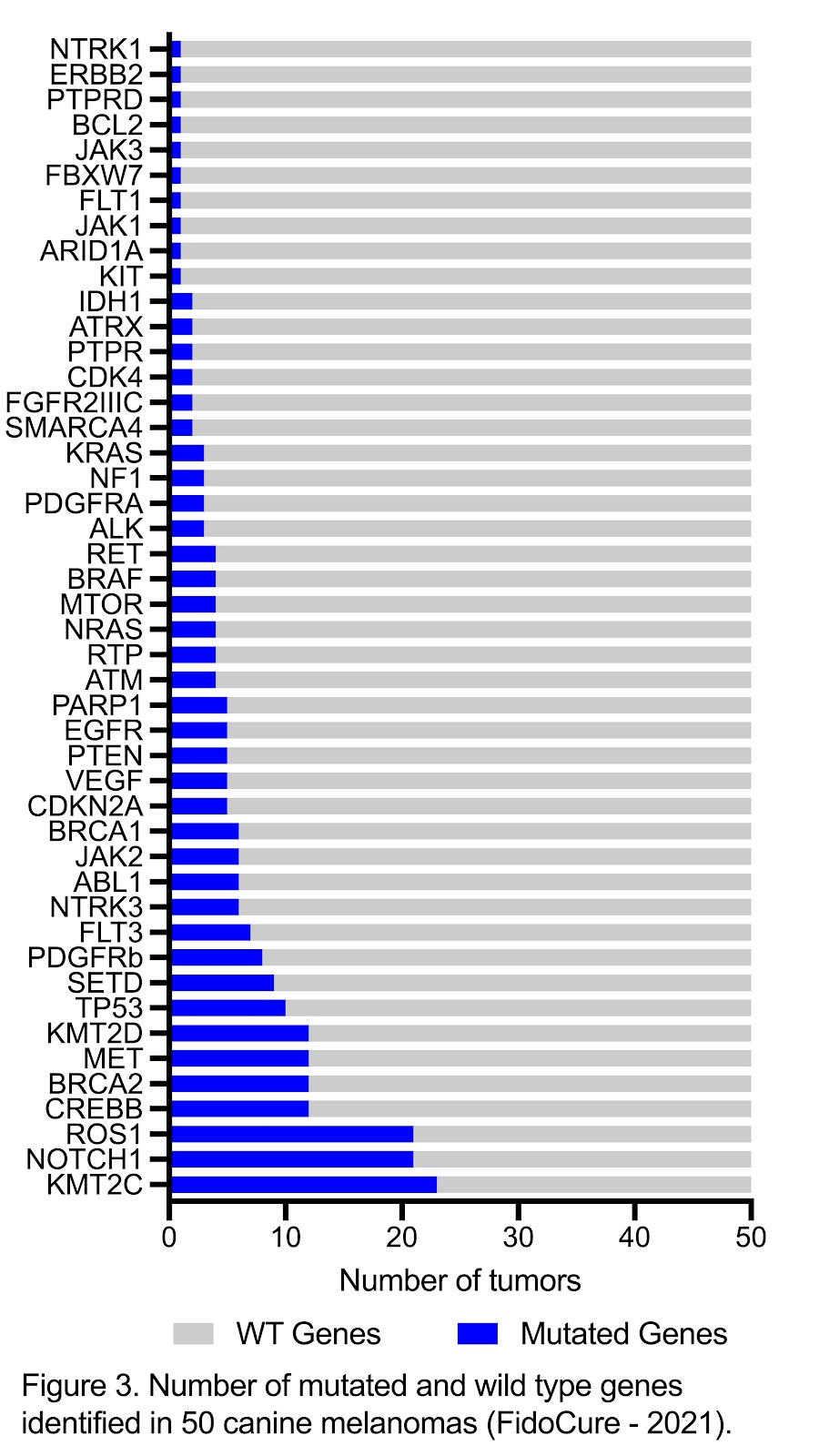
According to the literature, alterations in TP53 and PTEN genes are commonly associated with canine melanomas. Studies have shown that mutations in TP53 occur in 8% to 19% of melanomas, while mutations in PTEN are detected in 5% of tumors.26–28. These tumor suppressor genes are involved in cell cycle control, DNA repair, apoptosis, migration and growth. Mutations causing loss of function of these genes are related to cancer development in both humans and dogs6,29. In the FidoCure® database (Figure 3), mutations in TP53 and PTEN are observed in 20% and 10% of the canine melanomas, respectively. The RAS family consists of NRAS, KRAS and HRAS oncogenes that activate the MAPK pathway and induce cell proliferation30. Although NRAS and KRAS are not as commonly mutated in canine melanomas, as they are in human melanomas, targeting PI3K/AKT/mTOR and MAPK pathways with rapamycin and a MEK1/2 inhibitor allowed a synergistic effect on growth inhibition in canine and human melanoma cell lines 6, 30, 31.
Analysis of the FidoCure® database identified KMT2C and KMT2D genes mutated in 46% and 24% of tumors, respectively, being KMT2C the major mutated gene in these melanoma cases. The KMT2 family of genes promote genome accessibility and transcription in the epigenetic context. These genes are linked to tumorigenesis, mutagenesis and immune tolerance when mutated. KMT2C and KMT2D genes are altered with loss of function in approximately 27% of human melanomas. Therapeutic intervention to inhibit the effect of loss-of-function of tumor suppressor genes still represent a challenge, but recent discoveries of KMT2C and KMT2D roles in cancer cells have been stimulating different therapeutic strategies. The loss of KMT2D in a Zebrafish model for human Kabuki syndrome, for example, results in hyperactivation of MEK and RAS/MAPK pathway. This activation can increase the chance of tumor to respond to BRAF inhibitors representing a treatment possibility of KMT2D mutated tumors 32–35.
Both NOTCH1 and ROS1 were the next most commonly mutated genes according to the FidoCure database, with 42% of the melanoma cases having a mutation in NOTCH1 and 42% of the melanomas cases having a mutation in ROS1. Mutations in NOTCH1 are also identified in 60% of human melanomas and this genomic alteration contributes to tumor growth by affecting survival properties and leading to an immunosuppressive tumor microenvironment. High expression of NOTCH1 has been identified in endothelial cells of human melanomas and correlates with shorter progression-free survival41. The potential development of NOTCH1 inhibitors could potentialize the efficacy of immunomodulators and decrease tumor proliferation36–40.
The ROS1 gene encodes the receptor tyrosine kinase ROS1, which is one of the subfamily of insulin receptor genes and its protein functions as a growth or differentiation factor receptor in the cell. This gene is involved in several activation of signaling pathways such as RAS-ERK and JAK/STAT3 (cell survival and proliferation), PI3K/AKT/mTOR (cell survival and growth) and VAV3/RHO (cell migration and invasiveness by actin remodeling) 42–45. Tumors like human melanoma are observed expressing altered ROS1 gene, as also seen in canine melanomas in the FidoCure®database as a third most commonly mutated gene.
Canine melanomas represent a challenging tumor to treat in veterinary medicine, due to the aggressiveness, heterogeneity and resistance mechanisms of the melanoma cells. Although many different therapeutic strategies have been tried, the rapid and frequent development of metastatic lesions is a major factor causing survival times for dogs with malignant melanoma often to be shorter than 12 months. The incorporation of DNA sequencing in the diagnostic cascade for canine malignant melanomas, may reveal prognostic markers, potential therapeutic targets, and abnormally activated pathways. This information may facilitate the development of more effective therapeutic options for this aggressive and heterogeneous tumor.
References
1. Bolon, B., Calderwood Mays, M. B. & Hall, B. J. Characteristics of canine melanomas and comparison of histology and DNA ploidy to their biologic behavior. Vet. Pathol. 27, 96–102 (1990).
2. Prouteau, A. & André, C. Canine melanomas as models for human melanomas: Clinical, histological, and genetic comparison. Genes (Basel). 10, 1–20 (2019).
3. Hernandez, B. et al. Naturally Occurring Canine Melanoma as a Predictive Comparative Oncology Model for Human Mucosal and Other Triple Wild-Type Melanomas. Int. J. Mol. Sci. 19, 1–19 (2018).
4. Bosenberg, M., Arnheiter, H. & Kelsh, R. Melanoma in mankind’s best friend. Pigment Cell Melanoma Res. 27, 1 (2014).
5. Gillard, M. et al. Naturally occurring melanomas in dogs as models for non-UV pathways of human melanomas. Pigment Cell Melanoma Res. 27, 90–102 (2014).
6. Bergman, P. J., Selmic, L. E. & Kent, M. S. Melanoma. in Small Animal Clinical Oncology (eds. Vail, D. M., Thamm, D. H. & Liptak, J. M.) 367–381 (Elsevier, 2020).
7. Simpson, R. M. et al. Sporadic naturally occurring melanoma in dogs as a preclinical model for human melanoma. Pigment Cell Melanoma Res. 27, 37–47 (2014).
8. Bergin, I. L., Smedley, R. C., Esplin, D. G., Spangler, W. L. & Kiupel, M. Prognostic evaluation of ki67 threshold value in canine oral melanoma. Vet. Pathol. 48, 41–53 (2011).
9. Laprie, C. et al. MIB-1 immunoreactivity correlates with biologic behaviour in canine cutaneous melanoma. Vet. Dermatol. 12, 139–147 (2001).
10. Sarowitz, B. N., Davis, G. J. & Kim, S. Outcome and prognostic factors following curative-intent surgery for oral tumours in dogs: 234 cases (2004 to 2014). J. Small Anim. Pract. 58, 146–153 (2017).
11. Tuohy, J. L., Selmic, L. E., Worley, D. R., Ehrhart, N. P. & Withrow, S. J. Outcome following curative-intent surgery for oral melanoma in dogs:70 cases (1998–2011). J. Am. Vet. Med. Assoc. 245, 1266–1273 (2014).
12. Murphy, S. et al. Oral malignant melanoma - the effect of coarse fractionation radiotherapy alone or with adjuvant carboplatin therapy. Vet. Comp. Oncol. 3, 222–229 (2005).
13. Kawabe, M. et al. Outcomes of dogs undergoing radiotherapy for treatment of oral malignant melanoma: 111 cases (2006–2012). J. Am. Vet. Med. Assoc. 247, 1146–1153 (2015).
14. Cancedda, S. et al. Efficacy and side effects of radiation therapy in comparison with radiation therapy and temozolomide in the treatment of measurable canine malignant melanoma. Vet. Comp. Oncol. 14, e146–e157 (2016).
15. Liptak, J. M., Dernell, W. S., Rizzo, S. A. & Withrow, S. J. Partial foot amputation in 11 dogs. J. Am. Anim. Hosp. Assoc. 41, 47–55 (2005).
16. Boston, S. E. et al. Efficacy of systemic adjuvant therapies administered to dogs after excision of oral malignant melanomas: 151 cases (2001-2012). J. Am. Vet. Med. Assoc. 245, 401–407 (2014).
17. Tellado, M. N., Maglietti, F. H., Michinski, S. D., Marshall, G. R. & Signori, E. Electrochemotherapy in treatment of canine oral malignant melanoma and factors influencing treatment outcome. Radiol. Oncol. 54, 68–78 (2020).
18. Silveira, T. L. et al. Cyclooxygenase-2 expression is associated with infiltration of inflammatory cells in oral and skin canine melanomas. Vet. Comp. Oncol. 18, 727–738 (2020).
19. Seo, K. won et al. Antitumor effects of celecoxib in COX-2 expressing and non-expressing canine melanoma cell lines. Res. Vet. Sci. 96, 482–486 (2014).
20. Spugnini, E. P. et al. Pulse-mediated chemotherapy enhances local control and survival in a spontaneous canine model of primary mucosal melanoma. Melanoma Res. 16, 23–27 (2006).
21. Liao, J. C. F. et al. Vaccination with human tyrosinase DNA induces antibody responses in dogs with advanced melanoma. Cancer Immun. 6, 1–17 (2006).
22. Manley, C. A. et al. Xenogeneic Murine Tyrosinase DNA Vaccine for Malignant Melanoma of the Digit of Dogs. J. Vet. Intern. Med. 25, 94–99 (2011).
23. Bergman, P. J. Canine Oral Melanoma. Clin. Tech. Small Anim. Pract. 22, 55–60 (2007).
24. Verganti, S. et al. Use of Oncept melanoma vaccine in 69 canine oral malignant melanomas in the UK. J. Small Anim. Pract. 58, 10–16 (2017).
25. Bergman, P. J. Cancer Immunotherapies. Vet. Clin. North Am. Small Anim. Pract. 49, 881–902 (2019).
26. Poorman, K. et al. Comparative cytogenetic characterization of primary canine melanocytic lesions using array CGH and fluorescence in situ hybridization. Chromosom. Res. 23, 171–186 (2015).
27. Wong, K. et al. Cross-species genomic landscape comparison of human mucosal melanoma with canine oral and equine melanoma. Nat. Commun. 10, (2019).
28. Hendricks, W. P. D. et al. Somatic inactivating PTPRJ mutations and dysregulated pathways identified in canine malignant melanoma by integrated comparative genomic analysis. PLoS Genet. 14, 1–30 (2018).
29. Minami, A. et al. Cell Cycle Regulation via the p53, PTEN, and BRCA1 Tumor Suppressors. in New Aspects in Molecular and Cellular Mechanisms of Human Carcinogenesis (ed. Bulgin, D.) 53–66 (InTech, 2016). doi:10.5772/61116.
30. Fowles, J. S., Denton, C. L. & Gustafson, D. L. Comparative analysis of MAPK and PI3K/AKT pathway activation and inhibition in human and canine melanoma. Vet. Comp. Oncol. 13, 288–304 (2015).
31. Bowlt Blacklock, K. L. et al. Genome-wide analysis of canine oral malignant melanoma metastasis-associated gene expression. Sci. Rep. 9, 6511 (2019).
32. Fagan, R. J. & Dingwall, A. K. COMPASS Ascending: Emerging clues regarding the roles of MLL3/KMT2C and MLL2/KMT2D proteins in cancer. Cancer Lett. 458, 56–65 (2019).
33. Zhang, P. & Huang, Y. Genomic alterations in KMT2 family predict outcome of immune checkpoint therapy in multiple cancers. J. Hematol. Oncol. 14, 1–5 (2021).
34. Ali, M., Hom, R. A., Blakeslee, W., Ikenouye, L. & Kutateladze, T. G. Diverse functions of PHD fingers of the MLL/KMT2 subfamily. Biochim. Biophys. Acta - Mol. Cell Res. 1843, 366–371 (2014).
35. Tsai, I.-C. et al. Small molecule inhibition of RAS/MAPK signaling ameliorates developmental pathologies of Kabuki Syndrome. Sci. Rep. 8, 10779 (2018).
36. Qiu, H., Zmina, P. M., Huang, A. Y., Askew, D. & Bedogni, B. Inhibiting Notch1 enhances immunotherapy efficacy in melanoma by preventing Notch1 dependent immune suppressive properties. Cancer Lett. 434, 144–151 (2018).
37. Osawa, M. & Fisher, D. E. Notch and melanocytes: Diverse outcomes from a single signal. J. Invest. Dermatol. 128, 2571–2574 (2008).
38. Bedogni, B., Warneke, J. A., Nickoloff, B. J., Giaccia, A. J. & Powell, M. B. Notch1 is an effector of Akt and hypoxia in melanoma development. J. Clin. Invest. 118, 3660–3670 (2008).
39. Zhang, K. et al. A Notch1neuregulin1 autocrine signaling loop contributes to melanoma growth. Oncogene 31, 4609–4618 (2012).
40. Zhang, K. et al. Synchronized Targeting of Notch and ERBB Signaling Suppresses Melanoma Tumor Growth through Inhibition of Notch1 and ERBB3. J. Invest. Dermatol. 136, 464–472 (2016).
41. Wieland, E. et al. Endothelial Notch1 Activity Facilitates Metastasis. Cancer Cell 31, 355–367 (2017).
42. Drilon, A. et al. ROS1-dependent cancers — biology, diagnostics and therapeutics. Nat. Rev. Clin. Oncol. 18, 35–55 (2021).
43. Uguen, A. & De Braekeleer, M. ROS1 fusions in cancer: a review. Futur. Oncol. 12, 1911–1928 (2016).
44. Zong, C. S., Zeng, L., Jiang, Y., Sadowski, H. B. & Wang, L.-H. Stat3 Plays an Important Role in Oncogenic Ros- and Insulin-like Growth Factor I Receptor-induced Anchorage-independent Growth. J. Biol. Chem. 273, 28065–28072 (1998).
45. Zong, C. S., Chan, J. L. K., Yang, S.-K. & Wang, L.-H. Mutations of Ros Differentially Effecting Signal Transduction Pathways Leading to Cell Growth Versus Transformation. J. Biol. Chem. 272, 1500–1506 (1997).
46. Drilon, A. et al. Safety and Antitumor Activity of the Multitargeted Pan-TRK, ROS1, and ALK Inhibitor Entrectinib: Combined Results from Two Phase I Trials (ALKA-372-001 and STARTRK-1). Cancer Discov. 7, 400–409 (2017).